What is a Light Water Reactor? What is a Small Modular Reactor?
Nuclear reactor technology, old and new, explained.
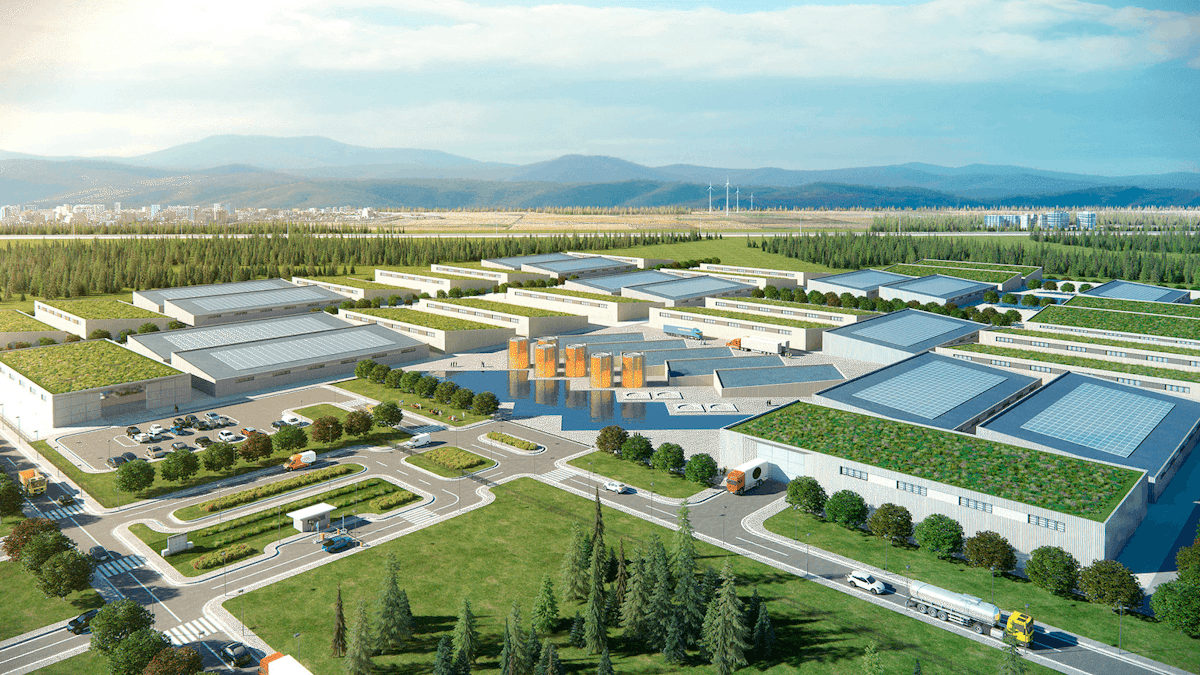
-
-
Share
-
Share via Twitter -
Share via Facebook -
Share via Email
-
“Light water” is a physics term for ordinary H2O. Reactors that use light water are referred to by that name because, in these designs, water—plain old water—does two important jobs.
One is to move heat. All nuclear reactors must capture the heat created when atoms are split and deliver it to where it can do useful work, like making electricity.
The other job is to “moderate” the speed of the neutrons, the sub-atomic particles that are thrown off when an atom is split, and that go on to break up other atoms. Almost all reactors need a way to slow down the neutrons, to a speed where they are more likely to split another atom’s nucleus when they hit it.
Around the world, most reactors use light water for those two functions. To be sure, light water is considerably cleaner than what comes out of a kitchen faucet (Good water chemistry prevents corrosion or buildup of minerals, allowing the reactor to run for many decades.) But that isn’t why it is called “light.” Physicists use the term to differentiate these ordinary water molecules from a rare second form of water molecule, called “heavy water.” The heavy water molecule also has one oxygen atom and two hydrogen atoms, but the hydrogen atoms are different: rather than a nucleus that is only one proton, each hydrogen of heavy water has a neutron and a proton (a form of hydrogen called “deuterium”). Heavy water is natural. It is not radioactive or toxic. But it literally weighs more.
Do all nuclear reactors use light water?
No. All the ones in commercial service in the United States do. But some reactors (including the Canadian Deuterium design, called Candu) use heavy water. A disadvantage of the light water reactor (often called an LWR) is that a water molecule sometimes steals a neutron from the nuclear chain reaction, and incorporates it into its hydrogen atom; in heavy water, the hydrogen atoms already have an extra neutron and are unlikely to absorb another. So it takes fewer neutrons to sustain the chain reaction in a heavy water reactor.
But obtaining heavy water requires processing huge amounts of seawater to find the stray molecules that incorporate deuterium. And engineers can easily use light water as the moderator and heat-transfer agent if they put the uranium fuel through an extra process, called enrichment, to change the mix of uranium types and increase the amount of the type that splits easily. The process of enrichment is key to several small modular reactor designs.
Other designs don’t use water in the reactor at all. Some use graphite, a pure form of carbon, as the moderator, and use a non-reactive gas, like helium, to carry the heat to a turbine. Some use salt that is heated until it takes a liquid form. Some reactors don’t use a moderator. These are called “fast” reactors because the neutrons are moving faster, and carrying more energy, a design trait that has several advantages.
But LWRs have been a popular choice because they have a design advantage: if the reactor overheats, the water boils away, and that stops the reaction. And early in the nuclear era, engineers preferred water because they were used to working with it in fossil-powered plants.
LWRs have been and still are very useful for making electricity. But they are less useful for making heat for industrial processes that now run on fossil gas, because, by industrial standards, steam from an LWR is at only a modest temperature. In a decarbonized economy, an efficient reactor fleet may be a mixture of LWRs and of other designs that can produce higher-temperature heat.
Do Small Modular Reactors use light water?
Some do, some don’t. The term “Small Modular Reactor,” or SMR, is a description of size, not reactor type. But some of the advanced designs that appear to be closest to commercial deployment are light water SMRs.
An SMR is usually defined as having an electricity output of 300 megawatts or less. This is “small” only in comparison to modern LWRs, which can have outputs four times higher. Fossil gas or coal plants in the range of 300 megawatts are common.
One reason for the buzz around SMRs is that they could be used to replace the boiler in a retiring coal plant, re-using the site’s existing grid connections, cooling water intake, transformers, and possibly even steam turbine.
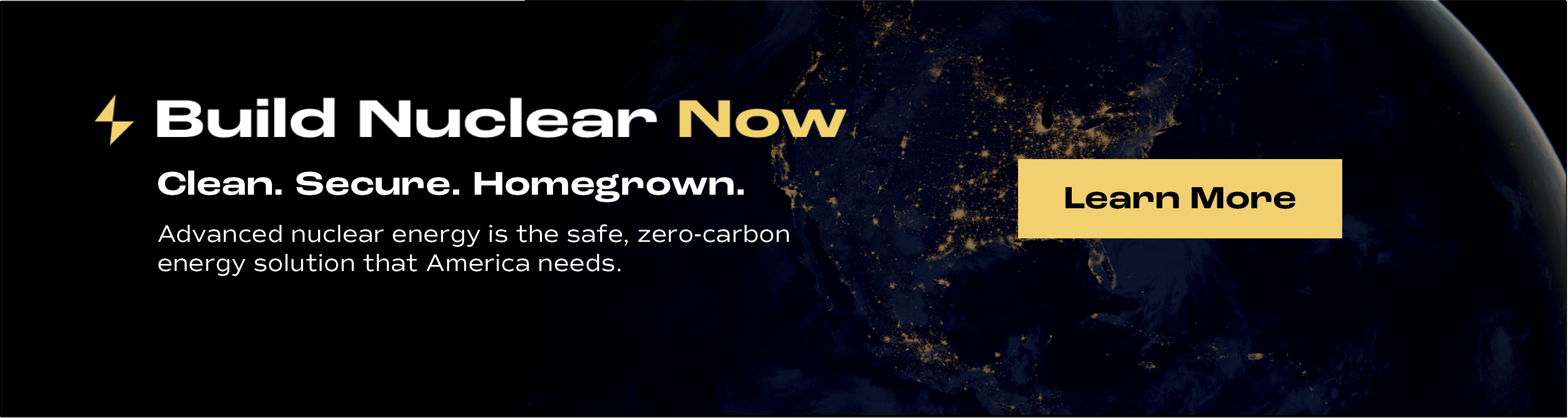
Some companies are working on “micro-reactors,” which are generally defined as 1 to 10 megawatts. These are generally not light-water designs. They may be extremely useful, especially for sites that are not on the grid or for sites that must operate even if the grid goes down, like military bases. Micro-reactors may play an important function and can displace diesel fuel, which is dirty and high-carbon. But they are unlikely to make up the bulk of a decarbonized power system because that would require deploying tens of thousands of them.
(Like “small,” the term “micro” could be misunderstood. A one-megawatt micro-reactor is powerful enough to run 1,000 window air conditioners.)
SMRs have inherent advantages. One is that they are small enough to be factory-built and shipped by truck to the power plant site, instead of built in the field. A factory that builds the same machine hundreds of times can do the work better and faster. Almost anything built in a factory costs less and is of higher quality than a complicated structure built piece-by-piece in the field.
In addition, power plants would be assembled, not built, a simpler, faster method. A factory could build SMR components while workers in the field poured concrete for the foundation and the buildings to house the reactors and other key components. Letting these two jobs overlap would shorten construction time. And that alone lowers costs. Given the vast scale of construction required to decarbonize the electric sector and increase electricity production enough to supply hundreds of millions of electric cars and trucks, and replace fossil fuel use for space heating, the speed of construction will be important.
Does the size of a nuclear reactor have any implications for safety?
Yes. A prime consideration in LWR design is reducing the possibility of fuel damage from overheating, because the fuel itself, a ceramic with a metal wrapper, is one of the barriers separating radioactive materials from the environment. Smaller cores are easier to cool, and several of the designs can assure cooling without any need to add water or to use electricity to run pumps or valves or to have the operators take action in the first few hours of an off-normal condition, or even in the first few days or weeks. This is a design criterion called “walk-away safe.” (Although it is a figure of speech; the operators would not actually walk away.)
In addition, SMRs designed in the twenty-first century can be built with security in mind, with key components located safely underground. Current reactors are highly secure, but require big staffs of guards to stay that way.
What is the status of Light Water SMRs today?
Among advanced reactors, light water SMRs are best able to make use of existing supply chains for fuel and components, and thus have a head start towards commercialization. They also make the best use of the existing high-voltage grid, which is important because getting approval for new transmission lines is very difficult.
A NuScale design has already been approved by the Nuclear Regulatory Commission.- An SMR design offered by GE-Hitachi mostly uses components already in use in other GE reactors; 80 percent of the components have NRC approval already.
Both the NuScale and GE-Hitachi designs, and some other designs, are able to change power output much more easily than the current generation of reactors, which is important for utilities that anticipate having to maintain reliability on a system with a lot of solar and wind generation, which are highly variable. Because the reactors can be “dispatched,” or ordered to produce at specified levels at specified hours, that makes light water SMRs attractive to utilities that have aggressive decarbonization goals but have not yet figured out how to achieve them.